Exploring the Invisible: The Role of Radiation Payload
17 June 2023 | DENIZ GÜneri
EXPLORING THE INVISIBLE: THE ROLE OF RADIATION PAYLOAD
17 JUNE 2023 | DENIZ GÜNERI
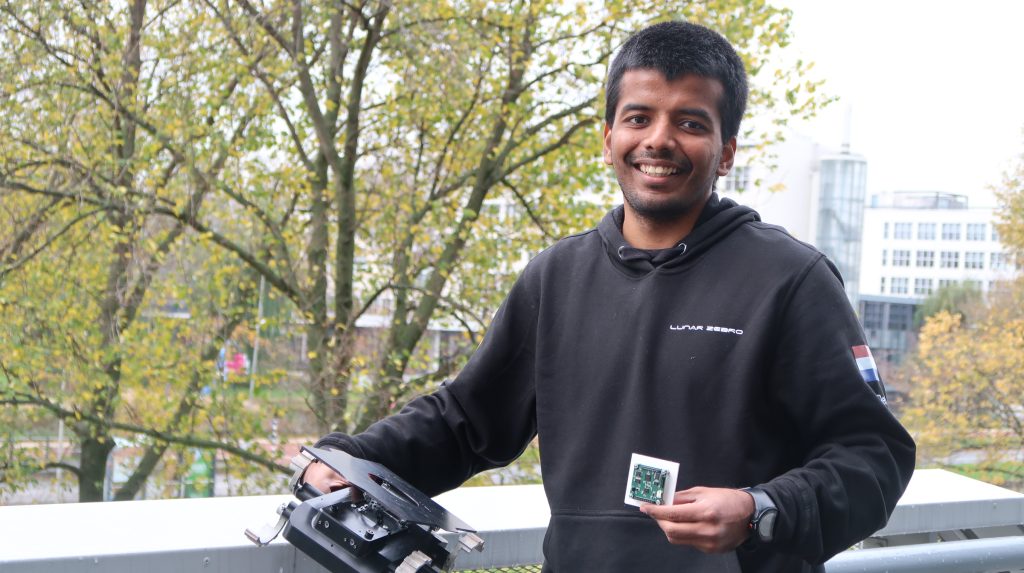
Ionizing Radiation does not hinder our daily lives on Earth very much. On the Moon, it is an entirely different story. Electronics, materials and living organisms require protection and adaptations to be able to survive in the lunar radiation environment. Therefore, the first step is to understand space radiation, which is the science mission of Lunar Zebro.
Sources for space radiation
The radiation we encounter in daily life -for example visible light, radio waves, ultraviolet light -do not possess enough energy to ionize electrons from atoms. This makes ionizing radiation fascinating to study because it interacts differently with matter than the radiation that we are used to in daily life.
Most space missions are strained by ionizing radiation from three main sources: the Sun, cosmic radiation and radiation trapped in magnetic fields. Firstly, the Sun emits Solar Energetic Particles (SEPs) in the form of the ever-present solar wind, as well as sporadic events like solar flares and coronal mass ejections (CMEs). The second type of radiation originates from high-energy events happening outside the solar system, such as supernovae explosions. Cosmic rays consist of high energy and charge (HZE) ions travelling at extremely high velocities in all directions. The last source is made up of radiation particles trapped in magnetic fields around planetary bodies and moons. Most notably, the trapping of solar protons and electrons by the Earth’s magnetosphere has led to the formation of the Van Allen Radiation Belts.
Effects of exposure to ionizing radiation
The main cause of concern from exposure to ionizing radiation stems from the undesirable effects that it can cause on living organisms, electronic components, and materials. Exposure to energetic particles can damage living tissue, lead to mutated genes and breakdown in various components of the microscopic bio-molecular machinery that drives life. On Earth, we are protected by the atmosphere and the magnetosphere, which attenuate, scatter, and trap the incoming high energy radiation. However, on planetary bodies such as the Moon, without an atmosphere or magnetosphere, ionizing radiation can reach the surface easily. A similar concern shows itself on Mars as well, as ionizing radiation concerns multiple planets with various concerns.
The effect of ionizing radiation with high linear energy transfer (LET) is akin to the impact of armor-piercing rounds; it causes some damage where it enters a material and even more as it travels through, depositing considerable amounts of energy along the way. This makes cosmic rays especially dangerous, even though they exhibit lower fluxes than solar energetic particles on average.
Models and measurements
Given the abundance of space radiation and its risks, a thorough understanding of the space radiation environment is crucial for carrying out crewed and robotic spaceflight activities in an effective, safe and sustainable manner. Therefore, it is important to understand the underlying behavior and functioning of the space radiation environment. We must be able to predict its evolution over time and space, as well as the resultant effects on space systems and biology. Currently, this is done through the development of theoretical models of radiation environments, and instruments that supply real world measurements. These help us refine the theoretical models and lower the amount of uncertainty in our predictions.
Conventional instruments and approaches
Most instruments that have been used so far for direct observation and measurement of space radiation are complex, bulky and generate copious amounts of sophisticated datasets. This often implies that these conventional instruments are costly to build and test. They require lengthy development timelines, which leads to them being deployed in space at infrequent intervals. Therefore, there are usually only a couple of operational instruments in any given region in deep space, at a certain point in time.
Partly due to the lack of observational data, the levels of inaccuracy or uncertainty in current models can be more than an order of magnitude. Due to the vastness of our solar system, let alone space, the acquisition of data with sufficient spatial and temporal resolution necessitates that more instruments are needed for effective and comprehensive measurement of the space radiation environment and other space weather effects. The characteristics of current instruments make this unfeasible.

LZ Radiation Payload
This is where a miniaturized, innovative instrument can come to the rescue, like the one for our Lunar Zebro rover. The ingredients that can make such innovative alternatives possible include trends that are seen under the “New Space” approach: the use of commercial-off-the-shelf (COTS) components, higher risk tolerance, engineering for modularity and harnessing the benefits of technological trends such as miniaturization.
Thesis work
In collaboration with Dr. Menicucci, Abhimanyu’s graduate research focused on three main aspects of development for the Lunar Zebro Radiation Payload.
To have an estimate of the nature of the ionizing radiation that would be encountered by the Radiation Payload on Lunar Zebro’s first voyage, tools such as ESA’s SPENVIS and NASA’s OLTARIS were used to predict the types and fluxes of particles and the effects of shielding provided by the rover’s body during various phases of the mission.
The engineering and development of the first working prototype of the Radiation Payload was an important milestone in the project. A design was created and then the physical electronic boards were built. The multi-disciplinary nature of the project meant that design, prototyping, and development activities involved elements of mechanical, electronics as well as software engineering, supported by a solid foundation of space systems engineering.
An integrated Floating Gate Dosimeter (FGDOS) lies at the heart of the Radiation Payload and functions as the core detector. Testing and calibration of the FGDOS was the most significant part of this project. This was done using proton beams of varying energies and intensities, which revealed the working characteristics of the sensor’s behavior. The Radiation Payload prototype’s performance and functioning were successfully demonstrated under various dose rates and beam energy levels. Experiments were conducted to observe how changes in temperature affect the measurements and how potentially undesirable effects could be compensated for. This included experiments which approximated mission conditions by subjecting the FGDOS to radiation and temperature change at the same time, which proved that the sensor data can be used after some post-processing, to derive accurate and reliable results.
Hence, the Lunar Zebro Science Payload, with its mission to analyze the lunar radiation environment, enables a new way of measuring and characterizing ionizing radiation in space.

Future applications
Anticipating that the first Lunar Zebro mission goes according to plan and that valuable scientific data is obtained from the Radiation Payload, much more ambitious goals await in the future. The next leap for Lunar Zebro is to fly swarms of rovers to the moon which can operate autonomously and enable unprecedented exploration and science. The elegance of this concept resides in how simple each unit of the swarm can be, while still leading to sophisticated collective behaviors and capabilities. Moreover, increased robustness that comes from having interchangeable and redundant rovers guarantees much higher chances of mission success. If each rover in the swarm is equipped with its own Radiation Payload, the swarm can be used for rapid spatial and temporal mapping of the space radiation environment. Such a novel capability, combined with the novel locomotion of Lunar Zebro can be used to gather environmental data, carry out scientific investigations, and explore the Moon like never before.

Afterword
To carry on the development and modelling of the Radiation Payload, the Lunar Zebro team is looking for bright and enthusiastic MSc students. If a chance to test and iterate the design of the payload making use of state-of-the-art irradiation facilities in the Netherlands and at CERN, Geneva appeals to you, be sure to check out the job description at – https://zebro.space/join/